Synthetic microbial consortia consisting of microorganisms with different synthetic genetic circuits or divided synthetic metabolic pathway components can exert functions that are beyond the capacities of single microorganisms. However, few consortia of microorganisms with different synthetic genetic circuits have been developed. We designed and constructed a synthetic microbial consortium composed of an enzyme-producing strain and a target chemical-producing strain using Escherichia coli for chemical production with efficient saccharification. The enzyme-producing strain harbored a synthetic genetic circuit to produce beta-glucosidase, which converts cellobiose to glucose, destroys itself via the lytic genes, and release the enzyme when the desired cell density is reached. The target chemical-producing strain was programmed by a synthetic genetic circuit to express enzymes in the synthetic metabolic pathway for isopropanol production when the enzyme-producing strain grows until release of the enzyme. Our results demonstrate the benefits of synthetic microbial consortia with distributed tasks for effective chemical production from biomass.
Genetic manipulation in cyanobacteria enables the direct production of valuable chemicals from carbon dioxide. However, there are still very few reports of the production of highly effective photosynthetic chemicals. Several synthetic metabolic pathways (e.g., isopropanol, acetone, isoprene, and fatty acids) have been constructed by branching from acetyl-CoA and malonyl-CoA, which are key intermediates for photosynthetic chemical production downstream of pyruvate decarboxylation. Recent reports of the absolute determination of cellular metabolites in Synechococcus elongatus PCC 7942 have shown that its acetyl-CoA levels corresponded to about one hundredth of the pyruvate levels. In short, one of the reasons for lower photosynthetic chemical production from acetyl-CoA and malonyl-CoA was the smaller flux to acetyl-CoA. Pyruvate decarboxylation is a primary pathway for acetyl-CoA synthesis from pyruvate and is mainly catalyzed by the pyruvate dehydrogenase complex (PDHc). In this study, we tried to enhance the flux toward acetyl-CoA from pyruvate by overexpressing PDH genes and, thus, catalyzing the conversion of pyruvate to acetyl-CoA via NADH generation. The overexpression of PDH genes cloned from S. elongatus PCC 7942 significantly increased PDHc enzymatic activity and intracellular acetyl-CoA levels in the crude cell extract. Although growth defects were observed in overexpressing strains of PDH genes, the combinational overexpression of PDH genes with the synthetic metabolic pathway for acetate or isopropanol resulted in about 7-fold to 9-fold improvement in its production titer, respectively (9.9 mM, 594.5 mg/L acetate, 4.9 mM, 294.5 mg/L isopropanol). PDH genes overexpression would, therefore, be useful not only for the production of these model chemicals, but also for the production of other chemicals that require acetyl-CoA as a key precursor.
γ-aminobutyric acid (GABA) is a drug and functional food additive and is used as a monomer for producing the biodegradable plastic, polyamide 4. Recently, direct GABA fermentation from glucose has been developed as an alternative to glutamate-based whole cell bioconversion. Although total productivity in fermentation is determined by the specific productivity and cell amount responsible for GABA production, the optimal metabolic state for GABA production conflicts with that for bacterial cell growth. Herein, we demonstrated metabolic state switching from the cell growth mode based on the metabolic pathways of the wild type strain to a GABA production mode based on a synthetic metabolic pathway in Escherichia coli through rewriting of the metabolic regulatory network and pathway engineering. The GABA production mode was achieved by multiple strategies such as conditional interruption of the TCA and glyoxylate cycles, engineering of GABA production pathway including a bypass for precursor metabolite supply, and upregulation of GABA transporter. As a result, we achieved 3-fold improvement in total GABA production titer and yield (4.8 g/L, 49.2% (mol/mol glucose)) in batch fermentation compared to the case without metabolic state switching (1.6 g/L, 16.4% (mol/mol glucose)). This study reports the highest GABA production performance among previous reports on GABA fermentation from glucose using engineered E. coli.
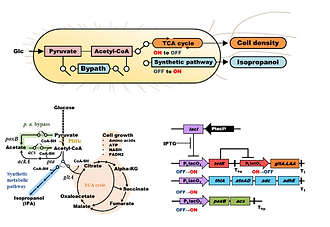
Almost all synthetic pathways for biofuel production are designed to require endogenous metabolites in glycolysis, such as phosphoenolpyruvate, pyruvate, and acetyl-CoA. However, such metabolites are also required for bacterial cell growth. To reduce the metabolic imbalance between cell growth and target chemical production, we previously constructed a metabolic toggle switch (MTS) as a conditional flux redirection tool controlling metabolic flux of TCA cycle toward isopropanol production. This approach succeeded to improve the isopropanol production titer and yield while ensuring sufficient cell growth. However, excess accumulation of pyruvate, the precursor for acetyl-CoA synthesis, was also observed. In this study, for efficient conversation of pyruvate to acetyl-CoA (pyruvate oxidation), we designed a synthetic metabolic bypass composed of poxB and acs with the MTS for acetyl-CoA supply from the excess pyruvate. When this designed bypass was expressed at the appropriate expression level associated with the conditional metabolic flux redirection, pyruvate accumulation was prevented, and the isopropanol production titer and yield were improved. Final isopropanol production titer of strain harboring MTS with the synthetic metabolic bypass improved 4.4-fold compared with strain without metabolic flux regulation, and it was 1.3-fold higher than that of strain harboring the conventional MTS alone. Additionally, glucose consumption was also improved 1.7-fold compared with strain without metabolic flux regulation. On the other hand, introduction of the synthetic metabolic bypass alone showed no improvement in isopropanol production and glucose consumption. These results showed that the improvement in bio-production process caused by synergy between the MTS and the synthetic metabolic bypass.
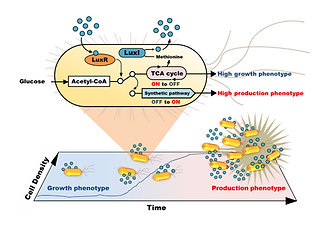
Metabolic Engineering
Chemicals production by engineered microorganisms often requires induction of target gene expression at an appropriate cell density to reduce conflict with cell growth. The lux system in Vibrio fischeri is a well-characterized model for cell density-dependent regulation of gene expression termed quorum sensing (QS). However, there are currently no reports for application of the lux system to microbial chemical production. Here, we constructed a synthetic lux system as a tunable cell density sensor-regulator using a synthetic luxpromoter and a positive feedback loop in Escherichia coli. In this system, self-induction of a target gene expression is driven by QS-signal, and its threshold cell density can be changed depending on the concentration of a chemical inducer. We demonstrate auto-redirection of metabolic flux from central metabolic pathways toward a synthetic isopropanol pathway at a desired cell density resulting in a significant increase in isopropanol production.
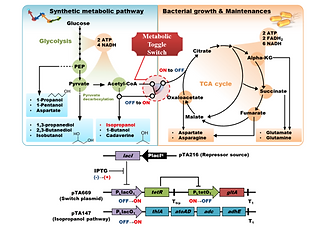
Metabolic Engineering
Overexpression of genes in production pathways and permanent knockout of genes in competing pathways are often employed to improve production titer and yield in metabolic engineering. However, the deletion of a pathway responsible for growth and cell maintenance has not previously been employed, even if its competition with the production pathway is obvious. In order to optimize intracellular metabolism at each fermentation phase for bacterial growth and production, a methodology employing conditional knockout is required. We constructed a metabolic toggle switch in Escherichia coli as a novel conditional knockout approach and applied it to isopropanol production. The resulting redirection of excess carbon flux caused by interruption of the TCA cycle via switching gltA OFF improved isopropanol production titer and yield up to 3.7 and 3.1 times, respectively. This approach is a useful tool to redirect carbon flux responsible for bacterial growth and/or cell maintenance toward a synthetic production pathway.
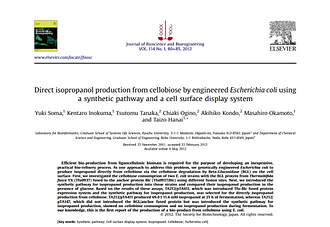
Journal of Bioscience and Bioengineering
Efficient bio-production from lignocellulosic biomass is required for the purpose of developing an inexpensive, practical bio-refinery process. As one approach to address this problem, we genetically engineered Escherichia coli to produce isopropanol directly from cellobiose via the cellobiose degradation by Beta-Glucosidase (BGL) on the cell surface. First, we investigated the cellobiose consumption of two E. coli strains with the BGL protein from Thermobifida fusca YX (Tfu0937) fused to the anchor protein Blc (Tfu0937/Blc) using different fusion sites. Next, we introduced the synthetic pathway for isopropanol production into those strains and compared their isopropanol production in the presence of glucose. Based on the results of these assays, TA212/pTA411, which was introduced Tfu-Blc fused protein expression system and the synthetic pathway for isopropanol production, was selected for the directly isopropanol production from cellobiose. TA212/pTA411 produced 69.0 ± 11.6 mM isopropanol at 21 h of fermentation, whereas TA212/pTA147, which did not introduced the BGL/anchor fused protein but was introduced the synthetic pathway for isopropanol production, showed no cellobiose consumption and no isopropanol production during fermentation. To our knowledge, this is the first report of the production of a bio-product from cellobiose using E. coli.